"A fact never went into partnership with a miracle. Truth scorns the assistance of wonders. A fact will fit every other fact in the universe, and that is how you can tell whether it is or is not a fact. A lie will not fit anything except another lie."
-Robert Green Ingersoll
One of the most amazing facts to comprehend about the Universe is that it actually is comprehensible! A few basic laws, properties and particles, given our current understanding, can take us from a hot, dense, nearly uniform Universe to the complexity of the billions of stars within the billions of galaxies we see today.
One of the most surprising, but most robust, of results from our investigation of the Universe is the fact that dark matter -- some form of matter that does not interact with light, with atoms, or with itself except gravitationally -- not only exists, but makes up about five times as much of the Universe as atoms do!
There is a whole suite of evidence that points towards this conclusion, but the two best pieces of evidence are the fluctuations in the cosmic microwave background,
and the distribution of galaxies -- known as large-scale structure -- throughout the Universe.
Now, the thing we'd like to do next, given what we presently know, is to figure out how to directly create and/or detect this dark matter, so we can find out exactly what it is!
Unfortunately, because it doesn't interact with normal matter except gravitationally, and the gravitational force is something like a factor of 1030 weaker than the other known forces, we haven't been able to do so, yet. (No disrespect to CRESST, DAMA or CoGeNT.)
But one of the questions we can ask that will have observable consequences is what kinetic energy this dark matter has relative to its mass!
The fluctuations in the microwave background, believe it or not, are completely insensitive to this! You could have dark matter that moves at ultrarelativistic speeds, or dark matter that barely moves at all, and the pattern of fluctuations wouldn't change at all.
But a number of things are sensitive to the speed of dark matter. As an analogy, think about it in terms of the molecules inside something like a balloon.
If the molecules are fast-moving, this corresponds to a high temperature, and the balloon would feel a significant pressure inside, and would therefore be large and the gas inside of it would be diffuse.
On the other hand, if the molecules are slow-moving, this corresponds to a low temperature, the balloon's pressure inside would be low and it would therefore be small, while the gas inside would be quite dense.
Now, if we apply this to dark matter, some parts of the analogy are no good. Molecules collide with one another and with the surface of the balloon; dark matter simply zips around with whatever speed it has in the expanding Universe. All it can do is either move slowly enough to help objects collapse gravitationally (and form stars, galaxies, clusters, etc.), or move quickly enough to hinder their formation.
So we look deep into the Universe's past to see what we have.
We see lots of structure far enough back in the past to place limits on how hot -- or fast-moving -- this dark matter is allowed to be. And we not only find galaxies and quasars when the Universe is just a few hundred million years old, although we do find that, and that's very important.
We find, on even tinier scales, collapsed clumps of primordial hydrogen gas that are incredibly dense and incredibly cold. Because of how deep and narrow those lines above are -- absorption lines of cold hydrogen clumps in the early Universe -- we can place very good constraints on how slow-moving dark matter needs to be.
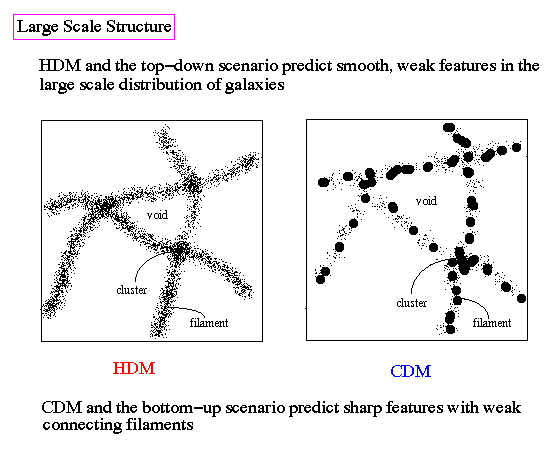
So we can rule out the picture known as hot dark matter, where something like a normal low-mass, relativistic neutrino makes up the bulk of the dark matter in our Universe.
But we can simulate different temperatures for this dark matter -- hot, cold, or somewhere in between -- and see what sort of predictions it gives.
Now, this is where it gets interesting. Both Warm and Cold dark matter give results that generally agree with our observations of small-and-large-scale structure. From scales that are about a hundred thousand light years and larger, these two types of dark matter form structure that are virtually indistinguishable. But when we look at the smallest scales, scales smaller than that of a single, large galaxy, that's where the differences are the most evident between the two theories.
One way to do this is to look is at individual galaxies themselves, and to reconstruct how the dark matter is distributed in them. Cold dark matter always gives predictions like the four upper lines, which are difficult to reconcile at their cores with observations. The isothermal model (the bottom line) has always given better fits, but has also always been chided as being ill-motivated, because there isn't a good particle candidate for it. (Unlike cold dark matter, which has WIMPs, axions, and a plethora of other candidates.)
But astronomers have been saying for a long time that if dark matter were warm, this fit would be much better. Physicists have preferred cold dark matter because of the possibilities of detection; indeed, that's where most of the efforts, including CDMS, XENON, Edelweiss, and the search at the LHC are sensitive.
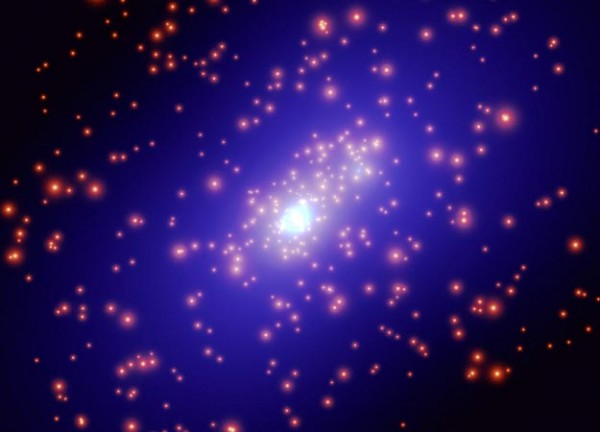
But there has long been another puzzle. On the scale of individual galaxies, like our Milky Way, cold dark matter predicts a very large number of small, clumpy satellite galaxies. And although we find many in our local group, there aren't nearly enough.
Well, Carlos Frenk at the University of Durham -- the "F" in the NFW profile from the earlier images -- has been working for the last few years on a set of very sensitive simulations involving dark matter and the formation of structure, and he's got a very important result.
Warm dark matter works! Dwarf galaxies around the Milky Way are less dense and less abundant than cold dark matter would give us, but the simulations using warm dark matter match up here, too!
And this is particularly amazing, because we can compare what cold dark matter and warm dark matter do, relative to one another, on the largest scales, where our observations have been very robust for a long time.
They're the same! On large scales, cold dark matter and warm dark matter give identical results for clustering; this is great, because this is where our theory matches up with observation the best!
But going to smaller and smaller scales?
Warm dark matter just works better. So if warm dark matter turns out to be the answer to our astronomy conundrums, what does this mean for the physics?
It means that we're not going to find dark matter where we're looking right now. Perhaps it's a sterile neutrino; a fourth family of neutrino that doesn't couple to the other ones the way we're accustomed to? Perhaps it's a new type of particle that we haven't considered before? Or perhaps it is something like an axion, only they aren't born either cold (like standard theory predicts) or hot (like a thermal relic would be), but warm, due to a new type of interaction or coupling?
Whatever the case may be, it's time to reopen the door for warm dark matter, and not to merely dismiss it because there are no satisfying particle candidates. Gravity and structure formation don't lie, so let's listen to what they're telling us!
- Log in to post comments
Another conundrum: brain scientists can't tell us where memories and thought process exist in the brain. A robust theory of consciousness will likely remain elusive. Conclusion: the universe, as a four manifold is a surface in a higher dimensional space.
Credible? What would this mean for the search for dark matter?
Very intriguing! And a very good walk-through that most people should be able to understand.
Does this have any bearing on the velocity of Dark Matter particles in the galactic halo, calculated to be a maximum of 8 kps?
DMs slow velocity in the halo was the reason why it was originally described as 'cold' (or so I've been led to believe).
Would you please provide a few easy to understand definitions of 'cold' 'warm' and 'hot' in the context of your post as the velocity definition normally used to determine DM to be 'cold' does not appear to valid according to your post.
Can you give rough temperature ranges for Cold and Warm in degrees K?
Would it be true that larger galaxies would have "warmer" clouds of dark matter associated with them than smaller galaxies?
Is the kinetic energy distribution of dark matter fixed?
Would any dark matter in the space between clusters be "hot" because any colder dark matter has been captured by a gravitational field?
Just a heads-up that comment #1 (by Bayan Escort) is a spam for some prostitution company in Istanbul. Feel free to remove this comment along with it, if you prefer.
TY, Tristan. #1 has been relegated to the spam filter. FYI, I get "only" about 30 new spam comments per day, so the filter actually does a pretty good job at this point!
Would a mix of "cold" and "hot" average out looking like "warm"?
Just wondering, while I'm familiar with relativistic speeds (not first-hand, of course!), I think they're generally considered to be around β>.1?, I have no idea how fast is considered 'ultrarelativistic'. What kind of velocity are we talking about here?
This is probably off base, but could it be that the value we have for the"speed of light in a vacuum" might be slightly off? Could interactions with virtual particle/antiparticle pairs slow down light rays as they travel through space, resulting in empty space having an index of refraction greater than one? Perhaps the non-interacting neutrinos just give a better approximation of a totally theoretical speed-of-light-in-a-vacuum.
Interesting thought, except that neutrinos are observed to change flavor. Observable change, of any kind, cannot occur at the exact speed c. (Note that c is a feature of spacetime itself. Despite its common name, it is not determined by light.)
Perhaps neutrinos travel slower than c not because of mass, but because they're being refracted by dark matter?
I'm curious how normal matter would appear from dark matter's perspective. Would it appear as cold, warm, or hot "dark matter"? Is it possible to compute an answer? I guess basically I'm asking what's the average kinetic energy of the universe's ordinary matter content.
how could it is possible that dark matter is infinite or there is no boundary for universe.
very good explanation ! A true translation from science language to "normal, human language".
thank you !
"DMs slow velocity in the halo was the reason why it was originally described as ‘cold’ (or so I’ve been led to believe)."
No, it was labelled cold because it isn't radiating any notable radiation, and as a black body radiator, this would be indication it is cold.: Stephan's Law. E= sigma T^4.
Something moving in bulk at velocity isn't hot, it's moving fast. Some bulk with a lot of internal unexpressed (externally) energy would be hot, not moving fast.
The air in the room is not moving at great speed, but the molecules trapped in there are moving internally at great speed, so you feel a temperature of around 20C. The air is warm. If you were to let molecules leave (and not be replaced), then it would cool down, because those molecules of air leaving the room are taking energy with them.