"In any case, the number three fitted perfectly the way quarks occur in nature." -Murray Gell-Mann
You might think that we know it all, at least as far as knowing-it-all is possible. After all, we know that matter is made up of atoms, which are made up of electrons and nuclei, and the nuclei are made up of protons and neutrons, and then the protons and neutrons are made up of quarks and gluons.
Along with the electrons, the quarks and gluons are -- as far as we know -- indivisible, which places them among the fundamental particles of the Universe.
Every proton and neutron is an example of a more general type of particle known as a baryon, which is a particle made up of three quarks, as well as the gluons that hold them together. Each quark has two types of charge: an electric charge, just like an electron has, as well as a color charge!
Unlike the electric charge, which is fixed for particles and has its force carried by a chargeless particle (the photon), a quark always carries a color -- either red, green or blue -- but that color always changes over time, since the gluons that carry the force are also colored!
Last year, I wrote up a brief introduction called The Strong Force For Beginners, which I encourage you to look at if you want more details about how this works. With six quarks and eight different gluons mediating the strong force, the number of different baryons, or stable/quasi-stable combinations of three quarks, are tremendous. The key is that, to exist, the combinations of quarks needs to be completely colorless when taken all together.
But baryons are not the only possibility for satisfying this.
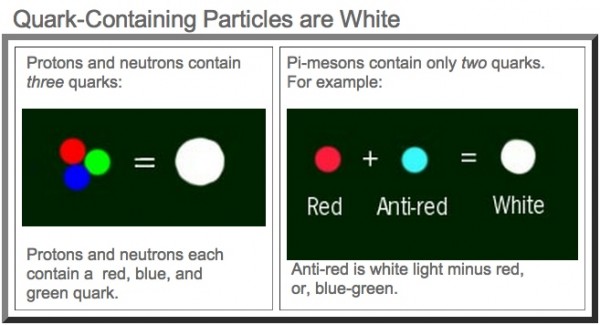
Each quark has a color charge, and each anti-quark has an anti-color charge, but these are not independent! For example:
- Anti-red is the same as blue+green, since red+anti-red or red+blue+green both = white.
- Anti-blue is the same as red+green, since blue+anti-blue or blue+red+green both = white.
- Anti-green is the same as blue+red, since green+anti-green or green+blue+red both = white.
So you can have a quark-antiquark combination, which is known as a meson. Or you could have three quarks, a baryon, or three antiquarks, an anti-baryon.
All of these particles, with the sole exception of the proton and antiproton, are unstable, and will decay over time.
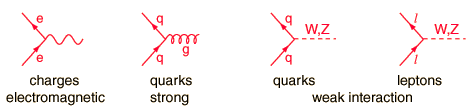
If a particle is unstable under the strong interactions, it will decay the most quickly, as the strong interaction is (duh) the strongest! A particle that undergoes a strong decay lives only some ~10-22 to 10-24 seconds.
If a particle is stable to the strong interactions but unstable to the electromagnetic interaction, it decays very quickly, but not quite as quickly as the strong interactions. A particle (like the neutral pion) that undergoes electromagnetic decay lives ~10-17 to 10-21 seconds.
And if a particle is both stable to the strong and electromagnetic interactions but not to the weak interactions -- which pretty means it needs to change its quark-type -- it lives the longest: ~10-8 to 10-13 seconds. (The lone exception is the free neutron, which for a variety of reasons lives about 10 minutes.)
But we can make other combinations of quarks and gluons than just mesons, baryons and antibaryons that are allowed by our governing theory: quantum chromodynamics, or QCD.
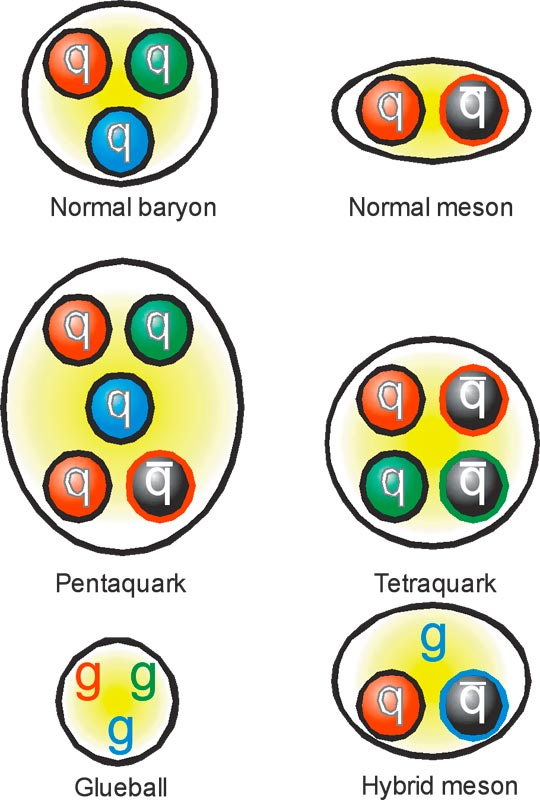
While they'll be unstable to strong decays, and hence will have incredibly short lifetimes, we can theoretically also have combinations such as tetraquarks (two quarks and two antiquarks), pentaquarks (four quarks and one antiquark, or four antiquarks and one quark), glueballs (bound states of gluons-only), or hybrid particles (a quasi-stable quark configuration with one or more extra gluons inside).
For a long time, these were only theoretical predictions of our theory of the strong interactions, but about a decade ago, claims started rolling in from particle accelerators that a pentaquark state had been discovered!
Now, it turns out that -- like many borderline discoveries in particle physics -- this one went away with more data, although there is at least one group that still claims to have found a pentaquark. (If you remember how long it took for physicists to confidently announce the discovery of the Higgs Boson, it's because they waited until they had enough data so that they could make sure they weren't announcing something that could possibly have gone away!)
And yet, states like tetraquark, pentaquarks and glueballs must exist if QCD is correct, so long as there are at least three colors.
In a surprising announcement earlier this week, two independent teams have just found overwhelming evidence for a tetraquark state: the Zc at a mass/energy of 3900 MeV! [Known, at least for now, as the Zc(3900).] Made up of two quarks, an up and a charm, and two anti-quarks, an anti-down and an anti-charm, this is the first confirmed particle made up of quarks-and-gluons that doesn't fit into our standard picture of either meson, baryon, anti-baryon, or a multi-baryon combination (which is what atomic nuclei are).
Yes, it's true that with a lifetime of less than 10-20 seconds, it's not like these particles have much effect on the Universe today. But back when the Universe was very young -- less than 100 picoseconds after the Big Bang -- these particles were just as abundant as any baryon or meson that existed, and provide the first real confirmation of one of the most novel predictions of our theory of the strong force! Here's hoping it continues to hold up, and that many more of these exotic particles await us in the future. (I'm particularly excited for the first glueball.) The search, and the journey, continues.
- Log in to post comments
As I understand it, which isn't a great deal, it is impossible for a quark to exist independently, but always must be glued to another quark, hence the term gluon?
@Tony Rotz: Basically, yes. The name "gluon" was coined back when physicists had a sense of humor, and didn't take their field quite so seriously as we do now :-/
Quarks can't be isolated from an existing bound state because the strong force (mediated by gluons) _increases_ with distance, rather than decreasing. (The converse, that the force decreases as the separation between quarks goes to zero, is called "asymptotic freedom").
If you want a classical analogy, take a strip of rubber in your hand. Label each end with a "Q" (for quark), and the rubber itself is the strong field (mediated by gluons). As you pull the ends apart, the rubber stretches, and just like a string exerts a stronger and strong restoring force. Eventually, you can pump enough energy in that the band breaks somewhere in the middle. Do you now have two free quarks? Nope! You have two bands, each one with two ends (go and label the new ends with "Q" as well). So from a single bound state (e.g., a meson), you now have two bound states, with shorter bands (and hence lower masses) than the original.
An interesting side note is that this argument really only applies to trying to "pull" a quark from an existing baryon or meson. It is conceivable that there are some left-over unbound quarks from the Big Bang. So far, searches for such entities passing through our partice-physics detectors have been unsuccessful.
As I understand it, the strong force doesn't become stronger at greater distances in an absolute sense -- as the separation becomes greater, the force between them does become less. It's that the relative strength of the force, the way it scales with 1/r^2, and compares with other forces at that distance, increases.
Here's an explanation: http://profmattstrassler.com/articles-and-posts/particle-physics-basics…
Could dark matter be "baryons" with non integer charge? An assemblage of quarks with a 2/3 or 4/3 charge couldn't interact with photons or charged particles because charge couldn't be conserved.
Electromagnetic interactions don't exchange or alter charge so how would charge not be conserved?
"Quarks can’t be isolated from an existing bound state because the strong force (mediated by gluons) _increases_ with distance, rather than decreasing."
The result being that if you stretch them apart further apart, at some point you've enough energy in the binding force to create a new pair of quarks that then bind to the two you're yanking at.
And you have now separated them and created some new baryons.
This ensures that the force has a maximum range based on the energies of the quarks that you are pulling apart.
The mechanism for this is that the force carrier becomes more and more energetic until the force carrier has the energy to decay into two products that just so happen to be the composites.
CB, the massless force carriers can reach infinite distance (because if they need to reach further, the force can create a smaller virtual particle as the force carrier without disobeying the uncertainty principle, therefore not have to decay into other particles that will be noticed and not therefore a force carrier.
Sort of.
So the range of these forces depend on the distribution of their attributing phenomenon. Mass or charge in the case of gravity and electromagnetism. And on large enough scales, this becomes neutral, therefore electromagnetic forces are limited by how much, and how large, a charged volume can be created.
Strong and weak nuclear force work over nm and therefore cannot collect a lot of their activating participant (color) because it just damn well won't fit in a volume that small.
Well, not since inflation.
So the strong force is short ranged. It DOES increase with distance, but only to a small distance. After that, the force carrier is de-virtualised into real particles with color and closes the gap, removing the color difference that you were trying to extend.
Google reader has been bringing me to this site for years.
will have to start learning some more about particle physics. Am a bit lost in all this.
Am pretty fine with cosmology.. things seem so elegant there. Particle physics is such a crowded, noisy jungle :D Sigh
So now I am confused. I thought tetra- et cetera resonances were suppressed instead of simply demanding higher energy, which is why we haven't seen them. But that would explain why 5.2 sigma (IIRC) was sufficient for some excitement instead of say, oh 7(?), for an unexpected observation.
Also, I read somewhere else that the di-meson configuration, which should be energetically preferred I take it, wasn't consistent with the decay times seen. Maybe it isn't because that the times given here seems to place it among meson decays, instead of first decaying into 2 mesons and then those decaying?
@ Wow:
Yeah, the distance Matt Strassler was talking about where the Strong Force becomes really strong was at a one proton-radius.
So if I understand your last paragraph, the short-range of the strong force is in part a consequence of gluons having color. When a real one is emitted, it ends up cancelling the color charge.
Could dark matter be “baryons” with non integer charge?
It turns out that any combination of quarks that satisfies the color charge neutrality condition must have a total electric charge which is an integer multiple of the electron charge. Single quarks have a charge of either +2/3 or -1/3, so the allowed charge states of three-quark combinations are +2, +1, 0, or -1. Multiply all of the above by -1 if you are talking about antiquarks. For mesons you can have +1, 0, or -1. Obviously, more options are possible if you allow more exotic states, but the electric charge will still add up to an integer multiple of the electron charge.
An assemblage of quarks with a 2/3 or 4/3 charge couldn’t interact with photons or charged particles because charge couldn’t be conserved.
Even if such assemblages could exist, the electromagnetic force is mediated by photons, which have charge 0. One of the vector bosons associated with the weak force also has 0 charge. Nothing prevents these entities from interacting by these paths. Furthermore, if we assume arguendo that a state with a charge of 4/3 must exist, then we can show that a state with a charge of 1/3 must also exist (e.g., if a uu combination exists, a ud combination must also exist), so interactions via the charged vector bosons are also allowed.
Pretty much, CB. Though the Feynman diagrams are more accurate. The explanation gives a decent framework to understand the process.
I never really got far with QCD. Never even read the Feynman lectures on that, never seemed to get round to getting them.
Thinking of it, maybe I ought to fire up and see if I can get them in ebook...
Thanks Michael K for the very understandable answer.
@CB #3 -- I read the page you linked to, and got rather confused. The author seems to assume that all forces are 1/r^2 with different coupling strengths, and that isn't true for either the strong or weak forces.
It's only true for EM and gravity because (a) the force carriers are massless (otherwise, you'd have a Yukawa coupling, exp(-r/L)); and (b) the force carriers do not interact with themselves/each other.
That second condition is what is violated in QCD (the gluons themselves carry color charge), and it is what leads to the "string-like" force (*NOT* "string theory", but the stretched-rubber-band model I described initially).
The fact that the force _increases_ with distance, is what makes all the bound hadrons so small. If you try to bind two quarks together over a distance larger than about 1 fm (the diameter of a nucleon) the gluon field ends up having so much potential energy that it spontaneously produces q-qbar pairs.
At that point, you end up with a pair of bound systems, each smaller than 1 fm. But inside that range, the binding force really does *increase* with distance.
But what about bigger "strongly bound" objects, like whole nuclei? The nucleons are bound to each other by the "strong force," but not directly by QCD gluons. Rather, you can sort of make an approximate analogy to molecules binding together with van der Waals forces -- each nucleon is tightly bound up with gluons, but there's a residual strong "color-static" field surrounding them, which allows them to attract and bind each other together. The typical model approach is to use virtual pions and rho mesons within the nucleus to provide a shortish-range Yukawa coupling.
Talking of rubber bands, take a look at the tricoloured trefoil here: http://en.wikipedia.org/wiki/Trefoil_knot#Nontriviality . Then starting from the bottom left, go round anticlockwise calling out the crossing-over directions: up down up. Then take a look at this topological quantum field theory web page: http://www.math.ist.utl.pt/~rpicken/tqft/ . Look at the top. Here's another TQFT web page: http://www.maths.ed.ac.uk/research/geom-top . The blue torus isn't a proton. That's an electron. Here's another one: http://scgp.stonybrook.edu/about . Always blue for some reason. I guess these guys talk. And they've seen the images:
http://www.opednews.com/articles/life_a_kathlyn__080309_electron_filmed…
http://io9.com/the-first-image-ever-of-a-hydrogen-atoms-orbital-struc-5…
@ Michael
I don't think he's assuming 1/r^2 drop-off; he's using that as the point of comparison for comparing force strength.
Beyond that I don't know; I've quite possibly misunderstood.
Could someone please explain why matter and anti-matter annihilate each other on contact (and do they really?) but quarks, anti-quarks, gluons and anti-gluons can exist peacefully in the same proton?
Thanks!
Colour separation.
You can have an top quark and an anti-up quark because the anti particle isn't the antiparticle of the positive quark.
And anti-proton consists of three quarks each of which is the antiparticle of one in the proton.
@Alissa: You generally don't have quarks and antiquarks together in the same baryon; it's either three quarks (such as uud, giving you a proton) or three antiquarks (such as anti-u + anti-u + anti-d), giving you an antiproton. With mesons, you have a quark and an antiquark, but they can be different flavors (such as u + anti-d). Where the quark and antiquark are of the same flavor, the meson in question is its own antiparticle, and it is invariably unstable.
Gluons are force mediators. Six of them have the effect of two quarks in a baryon exchanging their color charges, or the quarks in a meson going from A + anti-A to B + anti-B (where A and B are two of red, green, and blue); obviously three of them are the antiparticles of the other three. The other two (why there are two rather than three is above my pay grade since I am not a particle theorist) are color-neutral and act as their own antiparticles. (The photon and the Z0 vector boson are also their own antiparticles; the other two weak vector bosons are a particle-antiparticle pair.) When you have a gluon-antigluon pair simultaneously present in a proton, they are usually virtual particles, meaning that they coexist for a short time because energy and time obey a similar uncertainty relation as position and momentum.
Wow,
So then you wouldn't expect to observe a meson that is composed, for instance, of an up and an anti-up quark, right? (Or at least such a particle would be highly unstable) Or am I understanding you wrong?
Thanks Eric, I didn't see your post while I was writing my question. You've pretty much answered me.
No, though this is why this new baryon conglomeration was not expected (I hadn't noticed that five quarks were being included).
Elementary particle physics was really interesting, but I never really got the chance to keep up with it when I had to start working.
I could theorise about why this works, but it would be reasoning from analogy, and I suspect that there's been a lot of changes that I've been completely in the dark about in QCD.
It's a lot easier to be competent when understanding some other genius's work, but it's really not the same when you are at the cutting edge: there's still a hell of a lot of work to go to understand, and the path isn't an old and well-worn one.
Eric, I was assuming Alissa's query was "How come baryons like proton/anti-proton will collide and annihilate when they are each made of quarks, but quark and anti-quark don't".
It's a lot easier for charged particle/anti-particle, since the attraction increases as the particles get closer. But it's possible that conditionally stable results can be attained with a bound up/anti-up, since their attraction will fade as they close, and the presence of another colour may allow these near misses to be converted to a divergence.
But that is conjecture.
Wow: Yes that was my question, thanks. I still don't understand why the baryons (which are made of quarks) annihilate each other when the two colliding would be the quarks and anti-quarks inside them colliding; but the meson can exist using exactly the same (in theory) quarks and anti-quarks.
Eric: I did understand some of that, thanks! So you are saying colour plays a part in whether a quark and an anti-quark are truly explosive opposites? I must admit I'm still wrapping my brain around the quark/anti-quark combinations with the different "flavours" (up, anti-up etc.) to even think about the colours right now. Especially considering the mysterious colour switching process!
So, are you both annihilation only occurs if everything matches its opposite?
For example: A red up quark will only destroy a red anti-up quark? (or is that an anti-red anti-up quark? Goodness!)
Eric: I did understand some of that, thanks! So you are saying colour plays a part in whether a quark and an anti-quark are truly explosive opposites? I must admit I'm still wrapping my brain around the quark/anti-quark combinations with the different "flavours" (up, anti-up etc.) to even think about the colours right now. Especially considering the mysterious colour switching process!
Sorry about the last paragraph!
(Heh, I'm too busy to hang out here for a week or two, and look at all the cool stuff I missed;-)
For fellow laypeople, this quote from the Wikipedia article Ethan linked, is useful:
"The "color" of quarks and gluons is completely unrelated to visual perception of color.[1] Rather, it is a convenient (and somewhat whimsical) name for a property that has almost no manifestation at distances above the size of an atomic nucleus. The term color was chosen because the abstract property to which it refers has three kinds of values, which are analogized to the three primary colors of red, green, and blue.[2] The analogy lies in the fact that combination of three particles with red, green and blue color charges each doesn't interact with outside color charges (it is "white"). By comparison, the electromagnetic charge has only one kind of value. (Note that positive and negative electrical charges are the same kind of charge, they only differ by the sign. Color charges also can be negative. For example, "anti-red" color charge is simply a negative red color charge)."
http://en.wikipedia.org/wiki/Color_charge
For those of us who have internalized our understanding of electricity/electronics, this element is interesting: Electrical charge has a single value, "color" charge has three. That suggests a visual analogy: electrical charge has one axis of measurement, analogous to a straight line; "color" charge has three, analogous to three lines in the three spatial dimensions.
But this begs a question, though the question might even be "not even wrong" in the sense that it's asking the wrong question about the phenomenon: is there, or could there be, a technology application of the three values of color charge?
Lastly, it seems that the tetraquark can be accommodated within existing physics, since it was already predicted. Is that correct?, or are we looking at something that calls for a small piece of new physics to be added on?
Well, yes, a proton and a positron don't annihilate. They have to match up.
But the ant-Red is as equivalently made up of a positive Green and Blue. Therefore if there were a green or blue matching quark in there, then that anti-Red may become bound to the Green or Blue (or both!). Therefore never actually meet the Red to annihilate.
" is there, or could there be, a technology application of the three values of color charge? "
Almost 100% certainly not. The force range is very small and you can't collect a lot of one colour charge without making a whole slew of baryons and mesons as the energetically favoured decay product.